BORDER COLLIE
Coat Colours
Whilst the idea of the traditional Border Collie is still very much the glossy black dog with the white legs and feet, chest, collar, blaze and tip of the tail, it is becoming more well-known that this breed can come in quite a range of colours, patterns and markings. Colour is one of the first noticeable things about a dog and as such we now regularly receive enquiries and questions asking for recommendations of breeders for specific colours, however colour is usually quite low on the list of priorities for the majority of breeders behind the pup's overall health, temperament and conformation to the breed standard. We have created this resource to explain the genetics behind how those colours, patterns and markings are passed down through the breed, and which combinations can actually be quite risky - and we will also hopefully dispel some myths along the way!
As with baby humans, puppies inherit genes from their parents. The genes responsible for determining coat colour are a mixture of dominant and recessive genes. A puppy will inherit one copy of a gene from the sire (father) and one copy of a gene from the dam (mother). The distribution of these genes amongst a litter of puppies varies between individuals, so one litter can have a range of colours and markings dependent on the genetic combinations.
Firstly, when breeding it is important to note that whilst an understanding of colour genetics is helpful and important when breeding dogs who are/carry various colours, achieving a specific colour should be the last priority when planning a mating. The health, temperament and conformation to the breed standard are the most important considerations and as we will always reiterate - all colours of Border Collie are permissible for Dogs Australia registration and as such, colours are generally no longer rare so buyers beware of excessive prices - all puppies cost the same amount to raise, regardless of their colour.
Firstly, when breeding it is important to note that whilst an understanding of colour genetics is helpful and important when breeding dogs who are/carry various colours, achieving a specific colour should be the last priority when planning a mating. The health, temperament and conformation to the breed standard are the most important considerations and as we will always reiterate - all colours of Border Collie are permissible for Dogs Australia registration and as such, colours are generally no longer rare so buyers beware of excessive prices - all puppies cost the same amount to raise, regardless of their colour.
Genetics & Inheritance
We have created this resource to try and explain the basics behind Border Collie coat colour genetics in a way that both experienced breeders and new pet owners can read and understand, however simplifying complex scientific topics is not always easy. The content is quite lengthy in some sections by necessity. You may need to read through a few times or refer back to refresh your memory, however we have done our best to make the explanations and examples as easy to understand as possible, and occasionally repeat the information when giving examples or explaining further, to help tie it all together.
Melanin
All hair, skin and iris (eye) colour in humans and animals is due to a natural group of pigments broadly called melanin. Melanin is also responsible for the tanning of skin in sunlight. Likewise, the absence of melanin is responsible for albinism, where there is no pigment. There are five basic types of melanin: eumelanin, phaeomelanin, neuromelanin, allomelanin and pyomelanin. Eumelanin and phaeomelanin are the pigments that we will be concentrating on when we are talking about hair.
Melanin is produced in melanocytes, which are cells located throughout the body but in the case of hair, specifically at the base of the hair follicles. There is a gene named MC1R, which stores the instructions to build a protein called the Melanocortin 1 Receptor. This protein is usually located on the surface of the melanocytes and controls which type of melanin is produced. The type and amount of melanin determines hair colour, with eumelanin being responsible for black or brown hair, and phaeomelanin responsible for yellow/gold/red colouring. Both are present in all hair in various ratios determined by the genetic code in the dog's DNA. There are then other genes which control the production, intensity and appearance of these pigments and so in turn determine the exact coat colour and pattern of a dog.
Melanin
All hair, skin and iris (eye) colour in humans and animals is due to a natural group of pigments broadly called melanin. Melanin is also responsible for the tanning of skin in sunlight. Likewise, the absence of melanin is responsible for albinism, where there is no pigment. There are five basic types of melanin: eumelanin, phaeomelanin, neuromelanin, allomelanin and pyomelanin. Eumelanin and phaeomelanin are the pigments that we will be concentrating on when we are talking about hair.
Melanin is produced in melanocytes, which are cells located throughout the body but in the case of hair, specifically at the base of the hair follicles. There is a gene named MC1R, which stores the instructions to build a protein called the Melanocortin 1 Receptor. This protein is usually located on the surface of the melanocytes and controls which type of melanin is produced. The type and amount of melanin determines hair colour, with eumelanin being responsible for black or brown hair, and phaeomelanin responsible for yellow/gold/red colouring. Both are present in all hair in various ratios determined by the genetic code in the dog's DNA. There are then other genes which control the production, intensity and appearance of these pigments and so in turn determine the exact coat colour and pattern of a dog.
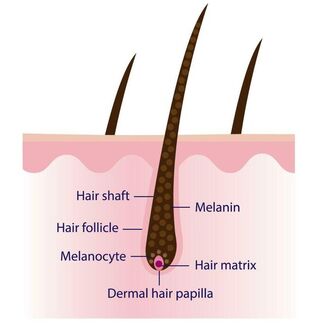
Diagram showing the basic anatomy of a hair follicle. The basic function of the dermal papilla is to stimulate the growth of the hair whilst the hair matrix creates the hair shaft and the melanocyte produces the melanin (pigment) that gives the hair shaft its colour. The colour itself will be determined by the Melanocortin 1 Receptor protein which is found on the surface of the melanocytes and will control the type and amount of melanin produced - eumelanin or phaeomelanin. This is then in turn influenced by other genes - see below.
Genes
Genes are segments of DNA on long, coiled up strands called 'chromosomes' and are responsible for storing the instructions required to build specific molecules, usually proteins within cells. Basically, the gene provides all of the information (code) required to build specific proteins, and the protein is the structural, functional and regulatory component of the cell. Each gene (DNA segment) is in a fixed location on the chromosome called a 'locus' with each locus being allocated a particular letter so that we know what they are referring to. If you think of the chromosome as a DNA neighbourhood where all the genes live, the locus is the street each particular group of genes is located on. There are always two chromosomes, one from the sire and one from the dam. They carry one copy of each gene that the parent carries at each locus and combine together to create a new genotype for the puppy. These copies, called 'alleles' (pronounced ah-leels), may be the same from each parent, or can be slightly different from each other and cause variations in the protein that is produced or expressed, which in turn changes how the trait is expressed. When two alleles match up, that combination gives us the exact information (description) we need to find that particular house (genotype) on that street (locus) in that neighbourhood (chromosome). The gene essentially controls the 'genotype' (the genetic makeup/code inherited from the parents - the design), and the protein controls the 'phenotype' (the actual physical expression or appearance - how it looks once it's built). Imagine the genotype for the front door of the house as written directions for ‘it will have a blue door’ and the phenotype being the blue door you see. The genotype is inherited from the parents and cannot be changed, whilst the phenotype is a result of the information from the genotype interacting with environmental factors and lifestyle. So the actual phenotype (physical appearance) of the front door may be faded blue with a big dent, which is a reflection of the genotype (it is blue), environmental factors (it is old paint in direct sunlight) and lifestyle (it has been damaged).
Genes are segments of DNA on long, coiled up strands called 'chromosomes' and are responsible for storing the instructions required to build specific molecules, usually proteins within cells. Basically, the gene provides all of the information (code) required to build specific proteins, and the protein is the structural, functional and regulatory component of the cell. Each gene (DNA segment) is in a fixed location on the chromosome called a 'locus' with each locus being allocated a particular letter so that we know what they are referring to. If you think of the chromosome as a DNA neighbourhood where all the genes live, the locus is the street each particular group of genes is located on. There are always two chromosomes, one from the sire and one from the dam. They carry one copy of each gene that the parent carries at each locus and combine together to create a new genotype for the puppy. These copies, called 'alleles' (pronounced ah-leels), may be the same from each parent, or can be slightly different from each other and cause variations in the protein that is produced or expressed, which in turn changes how the trait is expressed. When two alleles match up, that combination gives us the exact information (description) we need to find that particular house (genotype) on that street (locus) in that neighbourhood (chromosome). The gene essentially controls the 'genotype' (the genetic makeup/code inherited from the parents - the design), and the protein controls the 'phenotype' (the actual physical expression or appearance - how it looks once it's built). Imagine the genotype for the front door of the house as written directions for ‘it will have a blue door’ and the phenotype being the blue door you see. The genotype is inherited from the parents and cannot be changed, whilst the phenotype is a result of the information from the genotype interacting with environmental factors and lifestyle. So the actual phenotype (physical appearance) of the front door may be faded blue with a big dent, which is a reflection of the genotype (it is blue), environmental factors (it is old paint in direct sunlight) and lifestyle (it has been damaged).
Alleles
Alleles (different versions of the same genes at each locus) are usually (but not always) either dominant or recessive. A dominant allele creates a functional protein and only needs one copy from either parent to express that phenotype (characteristic), whilst a recessive allele creates a non-functional protein and requires the dog to have two identical copies (one from each parent) in order for that particular phenotype to be expressed. In a 'complete dominance' relationship between two alleles, when one of each allele is passed to a puppy, the phenotype of the dominant allele will be expressed, and the phenotype of the recessive allele will not, despite also being present. This is how recessive alleles can instead be 'carried' - one parent passes a copy of the allele to their offspring and because it is only one copy the phenotype cannot be expressed on that particular dog, however it can potentially still be passed on to their own offspring and be expressed when bred to a dog who also carries that allele.
Dominant alleles are indicated using a capital letter, while recessive alleles are listed using lower case. They are always listed as a pair - one copy from each parent. That pair of alleles is the genotype, the information for that exact phenotype written as two letters denoting what each allele in the pair represents. Two identical alleles in a genotype are referred to as 'homozygous' (homo = same) and two different alleles are 'heterozygous' (hetero = different). As such, the possible genotype combinations are as follows (using the black/brown colour gene as an example):
Alleles (different versions of the same genes at each locus) are usually (but not always) either dominant or recessive. A dominant allele creates a functional protein and only needs one copy from either parent to express that phenotype (characteristic), whilst a recessive allele creates a non-functional protein and requires the dog to have two identical copies (one from each parent) in order for that particular phenotype to be expressed. In a 'complete dominance' relationship between two alleles, when one of each allele is passed to a puppy, the phenotype of the dominant allele will be expressed, and the phenotype of the recessive allele will not, despite also being present. This is how recessive alleles can instead be 'carried' - one parent passes a copy of the allele to their offspring and because it is only one copy the phenotype cannot be expressed on that particular dog, however it can potentially still be passed on to their own offspring and be expressed when bred to a dog who also carries that allele.
Dominant alleles are indicated using a capital letter, while recessive alleles are listed using lower case. They are always listed as a pair - one copy from each parent. That pair of alleles is the genotype, the information for that exact phenotype written as two letters denoting what each allele in the pair represents. Two identical alleles in a genotype are referred to as 'homozygous' (homo = same) and two different alleles are 'heterozygous' (hetero = different). As such, the possible genotype combinations are as follows (using the black/brown colour gene as an example):
Locus |
B-Locus |
Allele |
B = Black (Dominant Allele) |
b = Brown (Recessive Allele) |
Genotype |
BB |
Bb |
bb |
Phenotype |
Homozygous Dominant Black |
Heterozygous Black |
Homozygous Recessive Brown |
Explanation |
Parents passed on two identical copies of the dominant allele. Dog is black and will only pass down black to offspring. |
Parents passed on two different alleles, the dominant allele decides the phenotype so the dog is black but has both copies and can pass either the black or brown allele down to offspring. |
Parents passed on two identical copies of the recessive allele. Dog is brown (chocolate) and will only pass down chocolate to offspring. |
Dominant & Recessive Inheritance
Dominant and recessive inheritance are useful concepts when it comes to trying to predict the likelihood of a litter inheriting certain things, especially genetic disorders, but the terms can be confusing when it comes to understanding exactly how a gene specifies a characteristic. This is partly because people observed dominant and recessive inheritance patterns before anyone knew anything about DNA and genes, or how genes pass on the information to the proteins that specify traits. Dominance describes the relationship between different alleles of a gene. A dominant trait usually corresponds to inheritance patterns that can be seen in a Punnett Square (demonstrated below). It does not indicate that a dominant trait may actually be 'strong', 'controlling' or 'better' to inherit, nor is a recessive allele 'weaker' or less desirable genetically. We know that if a dominant gene is present a recessive gene will usually not be expressed, however dominant alleles do not physically 'dominate' or 'repress' recessive alleles, they are simply easier to express since they only require one copy. Nor are dominant phenotypes always more common than recessive phenotypes - whilst recessive alleles do require two copies to be expressed, they can still be present in a population at a very high frequency. An example is human eye colour: eye colour is influenced mainly by two genes, with contributions from a few others. People with light eyes tend to carry recessive alleles of the major genes and people with dark eyes tend to carry dominant alleles - however most people in Scandinavia have light eyes, meaning the recessive alleles of these genes are much more common there than the dominant ones. This is a very broad human-centric example though - when it comes to breeding dogs, we are much more aware of the genetic makeup of the mating and therefore able to be much more selective about the pairing - with artificial insemination and appropriate international/interstate storage and transport more readily available we are seeing less geographical constraints and breeding programs are expanding to include more diverse lines.
At some locii, there are only two alleles where we can clearly identify which is dominant and which is recessive, and at others there are multiple alleles in a series which are then listed in a 'dominance hierarchy'. The most dominant allele will be expressed in the phenotype (physical appearance); a larger series of alleles may have multiple dominant and/or recessive alleles where one of the dominant alleles is dominant over another dominant allele, and one recessive allele is more recessive than another recessive allele. To distinguish them they are referred to as 'top dominants' and 'bottom recessives'. The K-Locus is an example of this where KB > Kbr > ky - we will discuss these more in-depth at K-Locus however it is a good example for our next points.
Complete Dominance vs Incomplete Dominance vs Co-Dominance
Making Predictions
With the table above it could also be easy to assume that dominant phenotypes (traits) are always more common than recessive, which could explain why we see far more black and white Border Collies than chocolate and white, however this is simply not always the case because most traits have complex, unpredictable inheritance patterns. We can make educated guesses, reasonably accurate predictions and plan litters with hope for particular coat colour outcomes, but aside from definite combinations that we know will have precise results, the final result of a litter from genetically diverse parents is usually down to chance because we simply cannot control the exact combination of alleles from each parent. When we talk about obtaining precise results, we are much better at controlling the outcomes we DON'T want, rather than obtaining the ones we do. In the case of breeding a litter hoping to produce merle, merle is a dominant gene so we know that breeding merle to merle will always produce merles, however it will also have a high chance of producing double merle (MM) puppies, which as we explain later puts the pups at a high risk of other health complications and therefore ethically it is an unacceptable risk (see M-Locus below). We also know that to produce a merle puppy they must have a copy of the dominant merle (M) allele; so, we know that one parent must be a merle. Only a single copy is required, we ethically cannot risk two copies, so the other parent will be the (mm) genotype and not carry merle. Remember, any copy of the dominant (M) allele will result in a merle coat, so this is the only possible ethical option. This however means that there is still no guarantee of merle puppies, and since it is a dominant gene meaning if it is present it is expressed, it also means that unless they are indeed merle themselves, none of those puppies will carry the merle gene either (because if they had it, they would BE it!).
A common tool for making predictions is the Punnett square (see below), which is a graphical representation of the possible genotypes for each puppy from a particular breeding, using the information we know about the parents. This would need to be repeated at every locus (location on the chromosome) and only shows the possibilities per puppy, not per litter. This is the same as predicting whether a baby will be a boy or a girl - we know there is a 50% chance of one or the other, but an accurate prediction is impossible, as is a prediction of the ratio of males and females within a litter. So, the likelihood of producing a particular colour does not change whether there is one puppy or ten - the genetic dice is rolled for every individual puppy as to what their genotype will be based on the possible combinations of their parents' alleles. The reason for this is because when breeding a dog they do not pass on both of their alleles to their offspring - there is only a 50% chance of one or the other for every reproductive cell (sperm or egg) produced, and that 50% is random. As you can see below, we have used Punnett squares to work out which colours are actually possible, and then the likelihood for each individual puppy to be a particular colour. Black is the dominant allele at the B-Locus shown as 'B' and chocolate is the recessive 'b'. Each box in the square equals a 1/4 possibility of producing a puppy with that exact genotype - in some litters two or more boxes predict a 50% or greater likelihood, but only with very specific combinations can a particular colour be absolutely guaranteed, and genetic testing is the only way to confirm the exact genotype of any dog with a dominant allele present (in this case black) who had a possible heterozygous combination (apart from the definite results of a BB and bb mating) - the reason being of course that if the dominant allele is present it will always be expressed, and so all dogs will be black and the only way to know if they carry brown (chocolate) or not before breeding is to test them and find out.
Dominant and recessive inheritance are useful concepts when it comes to trying to predict the likelihood of a litter inheriting certain things, especially genetic disorders, but the terms can be confusing when it comes to understanding exactly how a gene specifies a characteristic. This is partly because people observed dominant and recessive inheritance patterns before anyone knew anything about DNA and genes, or how genes pass on the information to the proteins that specify traits. Dominance describes the relationship between different alleles of a gene. A dominant trait usually corresponds to inheritance patterns that can be seen in a Punnett Square (demonstrated below). It does not indicate that a dominant trait may actually be 'strong', 'controlling' or 'better' to inherit, nor is a recessive allele 'weaker' or less desirable genetically. We know that if a dominant gene is present a recessive gene will usually not be expressed, however dominant alleles do not physically 'dominate' or 'repress' recessive alleles, they are simply easier to express since they only require one copy. Nor are dominant phenotypes always more common than recessive phenotypes - whilst recessive alleles do require two copies to be expressed, they can still be present in a population at a very high frequency. An example is human eye colour: eye colour is influenced mainly by two genes, with contributions from a few others. People with light eyes tend to carry recessive alleles of the major genes and people with dark eyes tend to carry dominant alleles - however most people in Scandinavia have light eyes, meaning the recessive alleles of these genes are much more common there than the dominant ones. This is a very broad human-centric example though - when it comes to breeding dogs, we are much more aware of the genetic makeup of the mating and therefore able to be much more selective about the pairing - with artificial insemination and appropriate international/interstate storage and transport more readily available we are seeing less geographical constraints and breeding programs are expanding to include more diverse lines.
At some locii, there are only two alleles where we can clearly identify which is dominant and which is recessive, and at others there are multiple alleles in a series which are then listed in a 'dominance hierarchy'. The most dominant allele will be expressed in the phenotype (physical appearance); a larger series of alleles may have multiple dominant and/or recessive alleles where one of the dominant alleles is dominant over another dominant allele, and one recessive allele is more recessive than another recessive allele. To distinguish them they are referred to as 'top dominants' and 'bottom recessives'. The K-Locus is an example of this where KB > Kbr > ky - we will discuss these more in-depth at K-Locus however it is a good example for our next points.
Complete Dominance vs Incomplete Dominance vs Co-Dominance
- Complete Dominance indicates the effect of one allele in a heterozygous (different alleles) genotype completely masking the effect of the other. The classic example of this as in the B/b example provided in the table above - the dog is either black, or it is brown. The black allele when present always masks the brown allele, and the dog is brown. This is the most simple version of dominant/recessive relationship, and many locii do work this way, but not all. There are also modifying genes at other locii which can influence how the genotype is expressed - but more on that later.
- Incomplete Dominance occurs where an allele that is usually known to be the dominant allele does not completely mask the effects of a recessive allele, with the result being a 'blending' of the two and exhibiting characteristics of both however will generally lean towards the characteristics of the dominant allele. Consider the K-Locus dominance hierarchy where KB > Kbr > ky. (KB) is solid colour and exhibits dominance over both other alleles, while (Kbr) is brindle and recessive to (KB) but dominant over (ky) which is the gene which allows sable or tanpoint/tricolour patterns to be expressed. The genotypes of KB/KB, KB/Kbr and KB/ky should all result in a solid coloured base coat. However we occasionally encounter coat colours in Border Collies that exhibit 'seal' or 'ghost tri' patterns, which appear to predominantly occur in KB/ky dogs. Despite the fact that (KB) as the dominant allele should not allow any pattern to be expressed on the coat, the dominance in this case seems to be 'incomplete' and allows the recessive (ky) allele to let the pattern 'leak through', and as such whichever pattern genotype the dog has at the A-Locus (either sable or tanpoint/tricolour) is being expressed, but not as clearly as it would be if (KB) was not present. So both alleles have instead blended and whilst tending towards the dominant solid colour, we can still see the pattern. This gives us 'seal' which is sable showing underneath the solid base colour, or 'ghost tri' which is tan points being barely visible. Seal on a black coat is sometimes also referred to as a 'bad black' - until an allele or modifier is discovered to identify why it occurs, it is generally considered an example of incomplete dominance of the (KB) allele.
- Co-Dominance differs from incomplete dominance in that it does not produce a 'blend' of the two traits, it instead clearly shows the two separate traits together. For example, red and white paint mixed together creates pink, so neither red nor white are completely dominant in that situation - however if we flicked red and white paint at a piece of paper we would end up with both red spots, and white spots - separate to each other but on the same piece of paper. This is co-dominance.
Making Predictions
With the table above it could also be easy to assume that dominant phenotypes (traits) are always more common than recessive, which could explain why we see far more black and white Border Collies than chocolate and white, however this is simply not always the case because most traits have complex, unpredictable inheritance patterns. We can make educated guesses, reasonably accurate predictions and plan litters with hope for particular coat colour outcomes, but aside from definite combinations that we know will have precise results, the final result of a litter from genetically diverse parents is usually down to chance because we simply cannot control the exact combination of alleles from each parent. When we talk about obtaining precise results, we are much better at controlling the outcomes we DON'T want, rather than obtaining the ones we do. In the case of breeding a litter hoping to produce merle, merle is a dominant gene so we know that breeding merle to merle will always produce merles, however it will also have a high chance of producing double merle (MM) puppies, which as we explain later puts the pups at a high risk of other health complications and therefore ethically it is an unacceptable risk (see M-Locus below). We also know that to produce a merle puppy they must have a copy of the dominant merle (M) allele; so, we know that one parent must be a merle. Only a single copy is required, we ethically cannot risk two copies, so the other parent will be the (mm) genotype and not carry merle. Remember, any copy of the dominant (M) allele will result in a merle coat, so this is the only possible ethical option. This however means that there is still no guarantee of merle puppies, and since it is a dominant gene meaning if it is present it is expressed, it also means that unless they are indeed merle themselves, none of those puppies will carry the merle gene either (because if they had it, they would BE it!).
A common tool for making predictions is the Punnett square (see below), which is a graphical representation of the possible genotypes for each puppy from a particular breeding, using the information we know about the parents. This would need to be repeated at every locus (location on the chromosome) and only shows the possibilities per puppy, not per litter. This is the same as predicting whether a baby will be a boy or a girl - we know there is a 50% chance of one or the other, but an accurate prediction is impossible, as is a prediction of the ratio of males and females within a litter. So, the likelihood of producing a particular colour does not change whether there is one puppy or ten - the genetic dice is rolled for every individual puppy as to what their genotype will be based on the possible combinations of their parents' alleles. The reason for this is because when breeding a dog they do not pass on both of their alleles to their offspring - there is only a 50% chance of one or the other for every reproductive cell (sperm or egg) produced, and that 50% is random. As you can see below, we have used Punnett squares to work out which colours are actually possible, and then the likelihood for each individual puppy to be a particular colour. Black is the dominant allele at the B-Locus shown as 'B' and chocolate is the recessive 'b'. Each box in the square equals a 1/4 possibility of producing a puppy with that exact genotype - in some litters two or more boxes predict a 50% or greater likelihood, but only with very specific combinations can a particular colour be absolutely guaranteed, and genetic testing is the only way to confirm the exact genotype of any dog with a dominant allele present (in this case black) who had a possible heterozygous combination (apart from the definite results of a BB and bb mating) - the reason being of course that if the dominant allele is present it will always be expressed, and so all dogs will be black and the only way to know if they carry brown (chocolate) or not before breeding is to test them and find out.
Border Collie Coat Colour Genetics
So now that we have a basic understanding of how genes are created and inherited, let's look more closely at the various coat colours, patterns and markings that we see in Border Collies specifically, on which locus they occur, and in turn how they look and interact with each other.
A-Z of Coat Colour Locii (Mostly)
A-Locus
(Agouti - Pigment Switching)
Affects distribution of both eumelanin and phaeomelanin.
Dominance Hierarchy: Ay > aw > at > a
The A-Locus controls the genes responsible for the distribution of melanin pigments, and is governed by a gene called ASIP, which produces what is known as the Agouti Signaling Protein. ASIP works alongside MC1R (mentioned earlier as the one that controls which type of melanin is produced by the melanocytes) to control red and black pigment switching in dogs. The melanocyte is stimulated by a hormone aptly named Melanocyte Stimulating Hormone produced in the pituitary gland. There are three types of MSH however with hair, it is the alpha Melanocyte Stimulating Hormone (α-MSH) that activates the production of the darker eumelanin, while ASIP instead activates the production of the yellow/red phaeomelanin. α-MSH and ASIP work in opposition to each other, and pigment switching is the name given to this change in whether the melanocyte produces eumelanin or phaeomelanin, causing bands of different colour along the length of a hair, or different colours in various places on the body. In Border Collies, this pigment switching results in the coat patterns we know as sable (light hairs with darker ends) and tricolour (blocks of lighter colour at certain points - eyebrows, cheeks, shoulders, elbows, knees and under the tail). Agouti is the term used for most wild type pigmentation found in many mammals such as wolves, rabbits and mice.
There are a number of different alleles at the A-Locus - the most widely accepted* listed in order of dominance are (Ay) fawn/sable, (aw) wolf sable and rarely seen in Border Collies but often seen in breeds such as German Shepherd Dogs, (at) tanpointed and (a) recessive black - this is rarely seen in any breed. Some Border Collies do however test as (aw) despite instead exhibiting the tan point pattern - it is possible that there exists another allele at the A-Locus that is as yet unknown and untested. Previously the sable allele was listed as (ay) however this was when dominant black was believed to be above it on the A-Locus - it has now been given its own locus (K) and sable was promoted to the most dominant in the series and gained a capital letter.
The most commonly seen in Border Collies are:
Ay - Dominant Yellow (Fawn/Sable)
at - Recessive Tanpointed (Tricolour)
Initially this seems quite straightforward, however unlike many of the other genotypes listed below at other locii this is not a simple case of the dominant allele completely expressing itself over the recessive. It has instead exhibited what is called incomplete dominance - homozygous (same) pairs will exhibit the phenotype as expected however a heterozygous (different) pair will mostly exhibit the dominant characteristics however also show some effect of the recessive allele as well.
Therefore, the possible genotypes and phenotypes that can be produced are as follows:
AyAy - Dominant Yellow - Clear Sable
Ayat - Heterozygous Sable - Shaded Sable* - the dominant 'sable' is expressed, but with darker fur in the coat
atat - Homozygous Tanpointed - Tanpointed (Tricolour)
*Progression in scientific research now means that we have been able to expand the series of alleles at the A-Locus and identify a further two alleles, Ays (shaded sable*) and 'asa' (saddle back). We expect that at some point with further research and testing we may see a change to this list of genotypes with the expansion of the allelic series to include 'Ays' and 'asa' and instead start to see that the genotypes for Shaded Sable may in fact be instead inclusive of 'Ays' as the dominant allele and exhibiting complete dominance as Shaded Sable rather than our previous belief that we were seeing incomplete dominance at play and expression of the recessive allele over what would otherwise have been a clear sable coat.
Both the sable and tricolour patterns can be expressed on any eumelanin base colour coat (listed below at B-Locus) however given that the (Ay) allele affects the extension of eumelanin similarly to the extension gene at the E-Locus, in the case of a Clear Sable (AyAy) the merle gene at the M-Locus will not be visible, so it is best to avoid a sable to merle mating (if we can't see that a dog is merle, it may mistakenly be bred to another merle and produce double merles). This does not mean that a good quality sable or red dog can never be bred to a good quality merle dog - all dogs should always be comprehensively breed panel DNA tested amongst other pre-breeding testing, which will confirm whether a sable or red dog is actually a merle or not.
It is thought that all Border Collies carry the genes for sable and/or tanpointed but their ability to express and produce these colours is dependent not only on the genotype combinations listed above, but also those at both the E-Locus and K-Locus. These three locii are so intertwined that it is important to understand their interaction with each other before we even really consider all other coat colour genetics. The K-Locus (Dominant Black) has the ability to effectively switch the Agouti genes on and off, while the E-Locus (Extension) can mask the colour of the dog and they will only ever express a golden red coat. In saying that, they can be some of the trickiest to understand and so we have kept the format on this page alphabetical to make it easy to mark your position, read ahead and then come back again if needed to fully understand their function.
Seal & Ghost Tri
Seal is a colour that is generally agreed to be linked to the A-Locus as dogs who present as seal generally test (AyAy) with (KBky) at the K-Locus (you can read more about this later), so are presumed to be a result of the A-Locus sable showing through their solid coat, as pictured below. Seal combinations can occur on all genotypes at B-Locus, D-Locus and M-Locus to create black, chocolate, blue, lilac seal and seal merle. This is similarly the case with 'Ghost Tri' where (atat) occurs alongside (KBky) and tanpoints show through very faintly on any of the above solid coats - so if it makes it easier to understand, you could consider seal a kind of 'Ghost Sable'. More research is required about how seal and ghost tri definitely occur as it is currently just a theory and as mentioned .in the 'incomplete dominance' section may instead be the result of an as-yet unidentified allele at the K-Locus, or a 'faulty' (KB) allele.
Saddle Pattern
It was previously thought that saddle pattern was caused by another allele on the A-Locus, however a gene known as RALY has been recently discovered to be a modifier of the (at) tanpoint gene. It is as such only visible on an (atat) dog that also does not have (KB) dominant black or (ee) at the E-Locus. Dogs exhibiting saddle pattern have one or two copies of this mutation which is testable. The modifier causes the eumelanin colour to retreat to the dog's back like a horse saddle, leaving the rest of the coat red.
There are a number of different alleles at the A-Locus - the most widely accepted* listed in order of dominance are (Ay) fawn/sable, (aw) wolf sable and rarely seen in Border Collies but often seen in breeds such as German Shepherd Dogs, (at) tanpointed and (a) recessive black - this is rarely seen in any breed. Some Border Collies do however test as (aw) despite instead exhibiting the tan point pattern - it is possible that there exists another allele at the A-Locus that is as yet unknown and untested. Previously the sable allele was listed as (ay) however this was when dominant black was believed to be above it on the A-Locus - it has now been given its own locus (K) and sable was promoted to the most dominant in the series and gained a capital letter.
The most commonly seen in Border Collies are:
Ay - Dominant Yellow (Fawn/Sable)
at - Recessive Tanpointed (Tricolour)
Initially this seems quite straightforward, however unlike many of the other genotypes listed below at other locii this is not a simple case of the dominant allele completely expressing itself over the recessive. It has instead exhibited what is called incomplete dominance - homozygous (same) pairs will exhibit the phenotype as expected however a heterozygous (different) pair will mostly exhibit the dominant characteristics however also show some effect of the recessive allele as well.
Therefore, the possible genotypes and phenotypes that can be produced are as follows:
AyAy - Dominant Yellow - Clear Sable
Ayat - Heterozygous Sable - Shaded Sable* - the dominant 'sable' is expressed, but with darker fur in the coat
atat - Homozygous Tanpointed - Tanpointed (Tricolour)
*Progression in scientific research now means that we have been able to expand the series of alleles at the A-Locus and identify a further two alleles, Ays (shaded sable*) and 'asa' (saddle back). We expect that at some point with further research and testing we may see a change to this list of genotypes with the expansion of the allelic series to include 'Ays' and 'asa' and instead start to see that the genotypes for Shaded Sable may in fact be instead inclusive of 'Ays' as the dominant allele and exhibiting complete dominance as Shaded Sable rather than our previous belief that we were seeing incomplete dominance at play and expression of the recessive allele over what would otherwise have been a clear sable coat.
Both the sable and tricolour patterns can be expressed on any eumelanin base colour coat (listed below at B-Locus) however given that the (Ay) allele affects the extension of eumelanin similarly to the extension gene at the E-Locus, in the case of a Clear Sable (AyAy) the merle gene at the M-Locus will not be visible, so it is best to avoid a sable to merle mating (if we can't see that a dog is merle, it may mistakenly be bred to another merle and produce double merles). This does not mean that a good quality sable or red dog can never be bred to a good quality merle dog - all dogs should always be comprehensively breed panel DNA tested amongst other pre-breeding testing, which will confirm whether a sable or red dog is actually a merle or not.
It is thought that all Border Collies carry the genes for sable and/or tanpointed but their ability to express and produce these colours is dependent not only on the genotype combinations listed above, but also those at both the E-Locus and K-Locus. These three locii are so intertwined that it is important to understand their interaction with each other before we even really consider all other coat colour genetics. The K-Locus (Dominant Black) has the ability to effectively switch the Agouti genes on and off, while the E-Locus (Extension) can mask the colour of the dog and they will only ever express a golden red coat. In saying that, they can be some of the trickiest to understand and so we have kept the format on this page alphabetical to make it easy to mark your position, read ahead and then come back again if needed to fully understand their function.
Seal & Ghost Tri
Seal is a colour that is generally agreed to be linked to the A-Locus as dogs who present as seal generally test (AyAy) with (KBky) at the K-Locus (you can read more about this later), so are presumed to be a result of the A-Locus sable showing through their solid coat, as pictured below. Seal combinations can occur on all genotypes at B-Locus, D-Locus and M-Locus to create black, chocolate, blue, lilac seal and seal merle. This is similarly the case with 'Ghost Tri' where (atat) occurs alongside (KBky) and tanpoints show through very faintly on any of the above solid coats - so if it makes it easier to understand, you could consider seal a kind of 'Ghost Sable'. More research is required about how seal and ghost tri definitely occur as it is currently just a theory and as mentioned .in the 'incomplete dominance' section may instead be the result of an as-yet unidentified allele at the K-Locus, or a 'faulty' (KB) allele.
Saddle Pattern
It was previously thought that saddle pattern was caused by another allele on the A-Locus, however a gene known as RALY has been recently discovered to be a modifier of the (at) tanpoint gene. It is as such only visible on an (atat) dog that also does not have (KB) dominant black or (ee) at the E-Locus. Dogs exhibiting saddle pattern have one or two copies of this mutation which is testable. The modifier causes the eumelanin colour to retreat to the dog's back like a horse saddle, leaving the rest of the coat red.
B-Locus
(Black/Brown)
Affects colour of eumelanin.
Dominance Hierarchy: B > b
Eumelanin is responsible for both black and chocolate coats, with a slight chemical difference in the bonds determining whether the dog has black eumelanin (B) or brown eumelanin (b). These alleles are located at the B-Locus on the chromosome and we have already looked at all the different genotypes that can exist at this locus in the Punnett square above. In other countries the brown colouring is often referred to as red - in Australia we call it chocolate.
BlackB-Locus: BB or Bb
Full Colour Black Dominant genotype; only one copy of the 'B' allele required, will always be expressed when present and always passed on. |
Brown (Chocolate)B-Locus: bb
Full Colour Brown Recessive genotype; will only be expressed when two 'b' alleles are present, but one copy can be passed on. |
D-Locus
(Dilute)
Affects intensity of eumelanin.
Dominance Hierarchy: D > d
The alleles at the D-Locus modify how the coat colour at the B-Locus is expressed - the D-Locus is where the alleles for dilution can be found. The dilute gene acts on eumelanin and is a recessive gene which modifies the expression of the pigment and affects the intensity of the colour. The two possible alleles are (D) - dominant full colour and (d) recessive dilute and the D-Locus holds part of the information needed to distribute pigment evenly in the hairs. If does not have this information the pigment will cluster together and dilute the appearance of the colour. Being a recessive gene two copies are required to produce the genotype, so any time that the dominant allele is present the dog will retain their full colour. This would be listed as either DD or Dd in the above black and chocolate dogs. When two recessive (d) alleles are present however, this genotype will cause a black coat to instead be expressed as blue, and a chocolate coat to be expressed as lilac.
BlueB-Locus: BB or Bb
D-Locus: dd Diluted Black Requires only one copy of the dominant 'B' allele but two copies of the recessive 'd' allele and will always pass on dilute. |
LilacB-Locus: bb
D-Locus: dd Diluted Brown Requires two copies of the recessive 'b' allele and two copies of the recessive 'd' allele and will always pass on both. |
E-Locus
(Eumelanin Extension)
Affects distribution of eumelanin.
Dominance Hierarchy: E > e
To understand how the alleles at the E-Locus function, we revisit the Melanocortin 1 Receptor we discussed right at the beginning of the genetics conversation and again at the A-Locus. This protein is the one that controls which type of melanin is produced by the melanocytes, whether it is eumelanin (black/brown) or phaeomelanin (yellow/gold/red). E-Locus holds the alleles responsible for producing eumelanin. The alleles found in Border Collies are (E) and (e). There is another (Em) which creates a dark 'mask' across the face of lighter coated breeds, however is not applicable here. If a dog has one copy of the dominant (E) allele, it can produce this pigment and therefore has the ability to express any of the four eumelanin coat colour phenotypes discussed above. When the MC1R gene is activated it stimulates the melanocytes to make eumelanin, however a mutation of the MC1R gene can cause the melanocytes to produce phaeomelanin instead - and this mutation is represented by the recessive (e) allele. As we know by now, one copy of the dominant allele (EE or Ee) gives the dog the ability to produce the 'dominant black' coat at the K-Locus (which could in actual fact end up as any variation on eumelanin be that black, brown or so on), however two copies of the recessive allele (ee) will instead result in the coat appearing red, regardless of the genotype at any other locus. They will still produce eumelanin and of course have a B-Locus genotype which will determine their 'real colour' alongside any additional modifying genes at other loci (such as merle, tricolour, sable etc.), but it is not visible. This is why the (ee) genotype is also referred to as the masking/melanistic masking gene (not to be confused with the (Em) masking allele), as it 'masks' or covers up the true colour and pattern of the dog by making them appear red. The true colour of the dog can be determined easily by genetic testing however the eumelanin in the skin can often solve the mystery - (BB) or (Bb) black dogs will have black noses, eye rims and lips, (bb) chocolate dogs will have liver. As with the (ay) allele at the A-Locus affecting the extension of eumelanin, in the case of an (ee) red dog the merle gene at the M-Locus will not be visible even if it is present, so it is best to avoid a red to merle mating (if we can't see that a dog is merle, it may mistakenly be bred to another merle and produce double merles). This does not mean that a good quality red or sable dog can never be bred to a good quality merle dog - all dogs should always be comprehensively breed panel DNA tested amongst other pre-breeding testing, which will confirm whether a red or sable dog is actually a merle or not.
There are many different descriptive names for the (ee) colour in Border Collies including blonde, champagne, cream, honey, golden, tan or wheaten, however all of these are just various intensities/shades of the same (ee) genotype. Internationally it is often referred to as 'Australian Red' or 'ee red' especially in those countries who refer to chocolate/brown as red - in Australia of course we just call it 'red'.
There are many different descriptive names for the (ee) colour in Border Collies including blonde, champagne, cream, honey, golden, tan or wheaten, however all of these are just various intensities/shades of the same (ee) genotype. Internationally it is often referred to as 'Australian Red' or 'ee red' especially in those countries who refer to chocolate/brown as red - in Australia of course we just call it 'red'.
H-Locus
(Harlequin)
Modifies merle.
Dominance Hierarchy: H > h
The harlequin gene located at the H-Locus is a merle modifier causing the grey background on a merle to be diluted to white and instead express as a white coat with patches of pigment, and this pattern (known as Dane Harlequin) is only recognised to occur in Great Danes, however it is worth listing here as there is often confusion over there also being such thing as a Harlequin Merle in Border Collies - with the location of this gene instead at the M-Locus. The Border Collie Harlequin Merle is not caused by a modifier, it is instead due to a variation in the actual merle gene (Mh), also called 'herding harlequin'. Read more at M-Locus.
K-Locus
(Dominant Black/Solid Colour)
Affects eumelanin, impacts on expression of A-Locus.
Dominance Hierarchy: KB > Kbr > ky
As mentioned at the A-Locus, all Border Collies are believed to carry the sable and/or tanpointed gene. You have also just learned that the E-Locus with the right combination of alleles is responsible for masking the true colour and pattern of the dog and expressing only phaeomelanin through a mutation of the MC1R gene - the K-Locus works by promoting eumelanin production and blocking both ASIP and α-MSH from binding to MC1R. This means that in effect, it can mask the agouti gene by stopping the pigment switching and producing only eumelanin, and the dog can therefore not express whatever pattern they have at their A-Locus and they will instead be a completely solid colour. This is referred to as Dominant Black but can be any base coat colour expressed via eumelanin. The A-Locus sable or tanpoint is dependent on the K-Locus to be able to either show its pattern or not, and the K-Locus is dependent on the E-Locus to be able to show its true colour or be covered by red. This makes the A, E and K-Locii the most important for determining the primary appearance of the dog, and the others then determine the secondary characteristics.
The series of alleles found at the K-Locus are (KB) Dominant Black, (Kbr) Brindle and (ky) Recessive Yellow/Non-Black. Their effects on the A-Locus are as follows:
KB - Completely blocks A-Locus pattern expression. Pigment cells will only produce eumelanin and the phenotype is known as 'dominant black', however any of the other base colours and variants that act on eumelanin can affect this so the dog will be a solid colour but this could be black, chocolate, blue, lilac or any of these in merle as well (as merle is not pigment switching, it is dilution). This is a dominant allele so when present will always be expressed (of course unless affected by the genotype at the E-Locus).
Kbr - Brindle allele does not pop up often in Border Collies, but when it does, this allele allows whatever is on the A-Locus to be expressed - with a brindle pattern over it. Sable therefore becomes brindle, and tanpointed becomes brindlepointed. This allele is dominant to (ky) but recessive to (KB). So it will not be expressed when paired with (KB), but will express itself when paired with (ky) and of course when it is a homozygous (KbrKbr) pair.
ky - This allele allows the agouti gene at the A-Locus to be expressed clearly without brindling, so this is where sable and tricolour dogs become able to express their markings. This allele is recessive and requires two copies to in turn allow expression of the A-Locus.
Therefore, the possible genotypes and phenotypes that can be produced are as follows:
The series of alleles found at the K-Locus are (KB) Dominant Black, (Kbr) Brindle and (ky) Recessive Yellow/Non-Black. Their effects on the A-Locus are as follows:
KB - Completely blocks A-Locus pattern expression. Pigment cells will only produce eumelanin and the phenotype is known as 'dominant black', however any of the other base colours and variants that act on eumelanin can affect this so the dog will be a solid colour but this could be black, chocolate, blue, lilac or any of these in merle as well (as merle is not pigment switching, it is dilution). This is a dominant allele so when present will always be expressed (of course unless affected by the genotype at the E-Locus).
Kbr - Brindle allele does not pop up often in Border Collies, but when it does, this allele allows whatever is on the A-Locus to be expressed - with a brindle pattern over it. Sable therefore becomes brindle, and tanpointed becomes brindlepointed. This allele is dominant to (ky) but recessive to (KB). So it will not be expressed when paired with (KB), but will express itself when paired with (ky) and of course when it is a homozygous (KbrKbr) pair.
ky - This allele allows the agouti gene at the A-Locus to be expressed clearly without brindling, so this is where sable and tricolour dogs become able to express their markings. This allele is recessive and requires two copies to in turn allow expression of the A-Locus.
Therefore, the possible genotypes and phenotypes that can be produced are as follows:
Genotype |
KBKB |
KBKbr |
KBky |
KbrKbr |
Kbrky |
kyky |
Phenotype |
Homozygous Dominant Black |
Heterozygous Dominant Black |
Heterozygous Dominant Black |
Homozygous Brindle |
Heterozygous Brindle |
Heterozygous Recessive Yellow |
Explanation |
Solid colour and will not be able to express anything at A-Locus. All offspring will only be a solid colour. |
Solid colour and will not be able to express anything at A-Locus however can pass either solid colour or brindle alleles to offspring. |
The dog should be a solid colour and should not be able to express anything at A-Locus*. Dogs can pass either allele to offspring. |
Whatever is at the A-Locus will be expressed with brindle instead of sable or tan, and brindle will be passed to all offspring. |
Whatever is at the A-Locus will be expressed with brindle instead of sable or tan. Both can be passed to offspring. |
Whatever is at the A-Locus will be expressed clearly. Two copies required to express and a copy will be passed down to all offspring. |
*As mentioned earlier, dogs exhibiting 'seal' or 'ghost tri' colouration often test (KBky ayay or KBky atat) which could be considered 'incomplete dominance' with sable or tricolour showing through a 'weaker' black (see A-Locus for more information).
M-Locus
(Merle)
Affects intensity of eumelanin.
Dominance Hierarchy: M > m
Merle is complex. This section has been broken up into basic merle genetics, double merles and advanced merle genetics, as it's important to understand the basics first.
Basic Merle Genetics
Like the dilute gene, the alleles at the M-Locus act on eumelanin and cause various grades of colour dilution rather than the full coat dilution of the D-Locus, which results instead in the 'patchy' merle pattern. It changes how the coat colour at the B-Locus and D-Locus are expressed, meaning merle can be appear on all four eumelanin pigmented coats - black, chocolate, blue and lilac. Some confusion often arises here, because when merle is expressed on a black coat the phenotype is referred to as a 'blue merle', however when it is expressed on a blue coat, it is instead referred to as a 'slate merle'. A common misconception is that a blue merle is the merle gene acting on a blue coat, which is not the case. The two basic alleles at the M-Locus on the chromosome are (M) - dominant merle and (m) recessive non-merle. Being a dominant gene only one copy is required to produce a merle genotype, so any time that the dominant allele is present the dog will express the merle phenotype (physical appearance).
Basic Merle Genetics
Like the dilute gene, the alleles at the M-Locus act on eumelanin and cause various grades of colour dilution rather than the full coat dilution of the D-Locus, which results instead in the 'patchy' merle pattern. It changes how the coat colour at the B-Locus and D-Locus are expressed, meaning merle can be appear on all four eumelanin pigmented coats - black, chocolate, blue and lilac. Some confusion often arises here, because when merle is expressed on a black coat the phenotype is referred to as a 'blue merle', however when it is expressed on a blue coat, it is instead referred to as a 'slate merle'. A common misconception is that a blue merle is the merle gene acting on a blue coat, which is not the case. The two basic alleles at the M-Locus on the chromosome are (M) - dominant merle and (m) recessive non-merle. Being a dominant gene only one copy is required to produce a merle genotype, so any time that the dominant allele is present the dog will express the merle phenotype (physical appearance).
Double Merles
A dog with two copies of the dominant allele (MM - homozygous merle) will be what is called a 'double merle'. This genotype is at risk of being produced any time two dogs with the dominant M allele are bred together. This is most often in the case in a merle-to-merle mating however there is also a risk where a homozygous (ee) genotype at E-Locus produces a red dog which is bred to a merle (see E-Locus). A double merle dog will inherit two copies of the dominant allele and it can cause a number of health issues including deafness, malformation of the eyes, vision impairment and infertility. Double merles will usually be quite easy to identify - they are often excessively white with very little colouring and abnormally small eyes. Due to these issues, it is ethically negligent to mate two merles as there is a 25% chance of producing this genotype and not worth the risk to the potential puppies. Despite a higher probability that the litter would produce merle puppies, there is still no guarantee and the risk of producing double merle puppies is such that no responsible breeder would ever plan this mating. The only way a litter could be absolutely guaranteed to produce merle puppies is if a double merle dog was bred from as they would then pass the dominant (M) allele to all offspring - however this situation would mean that the breeder was knowingly breeding a dog likely suffering from multiple health issues, with the only clear reason being profit.
A dog with two copies of the dominant allele (MM - homozygous merle) will be what is called a 'double merle'. This genotype is at risk of being produced any time two dogs with the dominant M allele are bred together. This is most often in the case in a merle-to-merle mating however there is also a risk where a homozygous (ee) genotype at E-Locus produces a red dog which is bred to a merle (see E-Locus). A double merle dog will inherit two copies of the dominant allele and it can cause a number of health issues including deafness, malformation of the eyes, vision impairment and infertility. Double merles will usually be quite easy to identify - they are often excessively white with very little colouring and abnormally small eyes. Due to these issues, it is ethically negligent to mate two merles as there is a 25% chance of producing this genotype and not worth the risk to the potential puppies. Despite a higher probability that the litter would produce merle puppies, there is still no guarantee and the risk of producing double merle puppies is such that no responsible breeder would ever plan this mating. The only way a litter could be absolutely guaranteed to produce merle puppies is if a double merle dog was bred from as they would then pass the dominant (M) allele to all offspring - however this situation would mean that the breeder was knowingly breeding a dog likely suffering from multiple health issues, with the only clear reason being profit.
Advanced Merle Genetics
Whilst merle is understood to be caused by just one allele (M), research has revealed a large variation in that allele leading to the designation of a number of new alleles. There are a number of ways in which gene mutations occur. One of these is through an extra section of DNA called a SINE insertion, which changes the way cells function - in the case of merle, the SINE (Short Interspersed Element) insertion impairs the ability of cells to produce normal pigment giving the patchy appearance. This mutation has three different 'body parts'; a head, a body and a tail. The original merle test only identified the body of the merle mutation and it was assumed that any length of tail would produce some kind of merle pattern, with the longer the tail the more merle expressed. When the test was introduced a number of solid coloured dogs were returning (MM) results - but this didn't make any sense, because the dogs clearly were not double merles.
Eventually the tail lengths started to be measured. They contained a long string of repeating base pairs, which was how their length was reported. Soon test results were given as (Mc) or 'cryptic' merle, with and a tail length of merle so short that it could no longer have an effect on the coat pattern. Soon however it started to become apparent that there was likely more than just (M) and (Mc) - and testing results started to show that there was a tail length longer than (Mc), but shorter than (M). This came to be known as (Ma) - 'atypical' merle. Testing began to identify the different base pair lengths of (M), (Ma), (Mc) and also included (Mh) - 'harlequin' merle. This has also been known as 'herding harlequin' and expresses similarly to the harlequin seen in Great Danes however all dogs were testing (hh) at the H-Locus for Non-Harlequin. (Mh) came back with base pair length longer than (M) and we now have seven recognised alleles at the M-Locus to not only identify merles, but also their differences. The longer the SINE insertion, the bigger the effect on the coat.
Whilst merle is understood to be caused by just one allele (M), research has revealed a large variation in that allele leading to the designation of a number of new alleles. There are a number of ways in which gene mutations occur. One of these is through an extra section of DNA called a SINE insertion, which changes the way cells function - in the case of merle, the SINE (Short Interspersed Element) insertion impairs the ability of cells to produce normal pigment giving the patchy appearance. This mutation has three different 'body parts'; a head, a body and a tail. The original merle test only identified the body of the merle mutation and it was assumed that any length of tail would produce some kind of merle pattern, with the longer the tail the more merle expressed. When the test was introduced a number of solid coloured dogs were returning (MM) results - but this didn't make any sense, because the dogs clearly were not double merles.
Eventually the tail lengths started to be measured. They contained a long string of repeating base pairs, which was how their length was reported. Soon test results were given as (Mc) or 'cryptic' merle, with and a tail length of merle so short that it could no longer have an effect on the coat pattern. Soon however it started to become apparent that there was likely more than just (M) and (Mc) - and testing results started to show that there was a tail length longer than (Mc), but shorter than (M). This came to be known as (Ma) - 'atypical' merle. Testing began to identify the different base pair lengths of (M), (Ma), (Mc) and also included (Mh) - 'harlequin' merle. This has also been known as 'herding harlequin' and expresses similarly to the harlequin seen in Great Danes however all dogs were testing (hh) at the H-Locus for Non-Harlequin. (Mh) came back with base pair length longer than (M) and we now have seven recognised alleles at the M-Locus to not only identify merles, but also their differences. The longer the SINE insertion, the bigger the effect on the coat.
m |
Mc |
Mc+ |
Ma |
Ma+ |
M |
Mh |
Non-Merle |
Cryptic Merle |
Cryptic Merle + |
Atypical Merle |
Atypical Merle + |
Standard Merle |
Harlequin Merle |
Length: |
200-230 |
231-246 |
247-254 |
255-264 |
265-268 |
269-280 |
The '+' indicates that there is a phenotypical (visible) difference but not enough to class it as a separate allele.
Cryptic Merle (Mc & Mc+)
No visible merle, but will test as (M) if using a lab that doesn't detect the advanced alleles. This is because they have the SINE insertion but it isn't long enough to affect their coat. If a homozygous (McMc) pair or with another merle allele some minor visible effects may be seen. A confirmed cryptic merle (Mc) dog is the ONLY safe merle to breed together or with other merles and can be considered non-merle (mm) however a (Mc+) may have a length long enough to have a slight effect on the coat and therefore should not be bred with a dog with a longer merle allele as it can result in the health issues seen in double merles.
Atypical Merle (Ma & Ma+)
Can range from no visible merle through to visible patterning. Some (MaMa) dogs can look like normal merles whilst others can have a seal-like dilute effect with minimal spots. Atypical merles should not be bred to merles with longer alleles to avoid potential double merle health issues.
Standard Merle (M)
The dog will have the visible classic merle pattern over its eumelanin - a diluted base colour and random patches of full colour.
Harlequin Merle (Mh)
Dogs with the (Mh) allele have the longest SINE insertion and therefore the most dramatic dilution effect on their coats, with most or all of the diluted base coat diluted further to white. Please note that this is not the same as the H-Locus harlequin (as listed earlier) - that is a merle modifier which could technically affect any merle allele, and whilst the visual effect is very similar, the (H) allele is only found in the Great Dane breed.
No visible merle, but will test as (M) if using a lab that doesn't detect the advanced alleles. This is because they have the SINE insertion but it isn't long enough to affect their coat. If a homozygous (McMc) pair or with another merle allele some minor visible effects may be seen. A confirmed cryptic merle (Mc) dog is the ONLY safe merle to breed together or with other merles and can be considered non-merle (mm) however a (Mc+) may have a length long enough to have a slight effect on the coat and therefore should not be bred with a dog with a longer merle allele as it can result in the health issues seen in double merles.
Atypical Merle (Ma & Ma+)
Can range from no visible merle through to visible patterning. Some (MaMa) dogs can look like normal merles whilst others can have a seal-like dilute effect with minimal spots. Atypical merles should not be bred to merles with longer alleles to avoid potential double merle health issues.
Standard Merle (M)
The dog will have the visible classic merle pattern over its eumelanin - a diluted base colour and random patches of full colour.
Harlequin Merle (Mh)
Dogs with the (Mh) allele have the longest SINE insertion and therefore the most dramatic dilution effect on their coats, with most or all of the diluted base coat diluted further to white. Please note that this is not the same as the H-Locus harlequin (as listed earlier) - that is a merle modifier which could technically affect any merle allele, and whilst the visual effect is very similar, the (H) allele is only found in the Great Dane breed.
S-Locus
(White Spotting)
Affects distribution of all pigment.
The S-Locus controls how much white a dog has. White on an otherwise coloured coat is called 'spotting', and the traditional white blaze, collar, chest, legs and tip of the tail that we see on most Border Collies is referred to as 'Irish White Spotting'. This pattern is very common and also seen on breeds such as Australian Shepherds, Bernese Mountain Dogs, Boston Terriers, Corgis, Shetland Sheepdogs and numerous others, however doesn't actually have a proven allele responsible for expressing it. There are two known alleles at the S-Locus, and two theoretical alleles. Before we get into those however, let's learn about white coats and how to get them in the first place.
Researchers located the S-Locus at the MITF gene, which plays a role in the development, survival and function of pigment cells. White is not a separate pigment - it is an absence of pigment altogether. We know that hair pigment is either black/brown eumelanin, or red/yellow phaeomelanin, and that these are produced by melanocytes. White is a result of no melanin at all, and mutations at the S-Locus affect the migration and survival of melanoblasts - the precursor cells for melanocytes. In turn this creates an absence of pigment cells in certain areas, the physical appearance being white markings. Further research is required to reliably identify and understand the reasons behind different spotting patterns (such as Irish White Spotting) however with the knowledge and theories we currently have, the following information has been generally accepted.
Known Alleles:
S - Dominant Solid Colour/No White Spotting - all cells are able to produce pigment.
sp - Recessive Spotting Piebald - there will be cells that can not produce pigment.
Theoretical Alleles:
si - Irish Spotting - the common Border Collie pattern of a white blaze, collar, chest, legs and tip of the tail, however less common overall in dogs than (sp).
sw - Extreme White - this has not been proven and so far all dogs with high white have been shown to be homozygous for (sp).
The spread of white usually follows the same theoretical rules - that is that white starts on the farthest 'edges' or 'trim' of the dog (the tip of the tail, muzzle, paws and sternum), then spreads to cover the muzzle, forehead, front of the chest, lower legs, more of the tail tip (the Irish Spotting pattern), then from the front to the back of the neck and further up the legs, until in the case of a Piebald dog only the head, back and tail base may still be coloured. From there, white will progress across the back, then the tail base and then the face markings, with the ears generally the last body parts to retain their colour. The theory behind this is that the dog retains pigment on the most important parts of the body (a loss of pigment in the hairs inside the ears will usually result in deafness for example).
There are obviously a number of different genotypes from combinations of the above alleles, which can lead to wide variation of white distribution in Border Collies. The most common and 'classic' phenotype is Irish Spotting, which we might assume would mean that many Border Collies would be homozygous for the (sisi) genotype however this is not yet reflected in genetic testing and many dogs even with the visible Irish spotting pattern still return (SS) or (Ssp) results. The Irish Spotting pattern usually breeds true and so two Irish Spotted dogs bred together will produce puppies with Irish Spotting and the white will likely not increase. We can expect that a solid coloured dog bred to an Irish Spotted dog will produce less white (receding in the reverse of the 'rule' of white spread back towards the 'trim'), whilst an Irish Spotted dog bred to a dog carrying the Piebald gene will produce a higher amount of white. This is what we could expect is the case in dogs with more white around the neck or undersides of the body.
Homozygous Piebald (spsp) usually produces a coloured head, base of the tail and patches on the body, and is often referred to as being 'white factored', which is a term sometimes used for a dog with more than the 'normal' amount of white expected in the classic Irish Spotting markings. The amount of white can vary widely, however in the case of Extreme White dogs (as mentioned previously all are currently testing as (spsp) just like regular Piebalds, there are just small amounts of colour on the head, and sometimes at the base of the tail or on the body. The nose is sometimes pink or partly pink and the eyes may be blue. The large difference between Piebald and Extreme White dogs is what has led to the theory that the (sw) allele exists, however it is not yet proven. Extreme white should be avoided and so the breeding of two white factored/piebald dogs together should always take into account how much white they have and the likely outcome of the breeding. For this reason, they should also not be bred to merle, as the combination of increased white at the S-Locus and random pigment deletion at the M-Locus would increase the chance of hearing and sight deficiencies.
Researchers located the S-Locus at the MITF gene, which plays a role in the development, survival and function of pigment cells. White is not a separate pigment - it is an absence of pigment altogether. We know that hair pigment is either black/brown eumelanin, or red/yellow phaeomelanin, and that these are produced by melanocytes. White is a result of no melanin at all, and mutations at the S-Locus affect the migration and survival of melanoblasts - the precursor cells for melanocytes. In turn this creates an absence of pigment cells in certain areas, the physical appearance being white markings. Further research is required to reliably identify and understand the reasons behind different spotting patterns (such as Irish White Spotting) however with the knowledge and theories we currently have, the following information has been generally accepted.
Known Alleles:
S - Dominant Solid Colour/No White Spotting - all cells are able to produce pigment.
sp - Recessive Spotting Piebald - there will be cells that can not produce pigment.
Theoretical Alleles:
si - Irish Spotting - the common Border Collie pattern of a white blaze, collar, chest, legs and tip of the tail, however less common overall in dogs than (sp).
sw - Extreme White - this has not been proven and so far all dogs with high white have been shown to be homozygous for (sp).
The spread of white usually follows the same theoretical rules - that is that white starts on the farthest 'edges' or 'trim' of the dog (the tip of the tail, muzzle, paws and sternum), then spreads to cover the muzzle, forehead, front of the chest, lower legs, more of the tail tip (the Irish Spotting pattern), then from the front to the back of the neck and further up the legs, until in the case of a Piebald dog only the head, back and tail base may still be coloured. From there, white will progress across the back, then the tail base and then the face markings, with the ears generally the last body parts to retain their colour. The theory behind this is that the dog retains pigment on the most important parts of the body (a loss of pigment in the hairs inside the ears will usually result in deafness for example).
There are obviously a number of different genotypes from combinations of the above alleles, which can lead to wide variation of white distribution in Border Collies. The most common and 'classic' phenotype is Irish Spotting, which we might assume would mean that many Border Collies would be homozygous for the (sisi) genotype however this is not yet reflected in genetic testing and many dogs even with the visible Irish spotting pattern still return (SS) or (Ssp) results. The Irish Spotting pattern usually breeds true and so two Irish Spotted dogs bred together will produce puppies with Irish Spotting and the white will likely not increase. We can expect that a solid coloured dog bred to an Irish Spotted dog will produce less white (receding in the reverse of the 'rule' of white spread back towards the 'trim'), whilst an Irish Spotted dog bred to a dog carrying the Piebald gene will produce a higher amount of white. This is what we could expect is the case in dogs with more white around the neck or undersides of the body.
Homozygous Piebald (spsp) usually produces a coloured head, base of the tail and patches on the body, and is often referred to as being 'white factored', which is a term sometimes used for a dog with more than the 'normal' amount of white expected in the classic Irish Spotting markings. The amount of white can vary widely, however in the case of Extreme White dogs (as mentioned previously all are currently testing as (spsp) just like regular Piebalds, there are just small amounts of colour on the head, and sometimes at the base of the tail or on the body. The nose is sometimes pink or partly pink and the eyes may be blue. The large difference between Piebald and Extreme White dogs is what has led to the theory that the (sw) allele exists, however it is not yet proven. Extreme white should be avoided and so the breeding of two white factored/piebald dogs together should always take into account how much white they have and the likely outcome of the breeding. For this reason, they should also not be bred to merle, as the combination of increased white at the S-Locus and random pigment deletion at the M-Locus would increase the chance of hearing and sight deficiencies.
T-Locus
(Ticking)
Controls flecks of pigment on otherwise unpigmented (white) areas.
Dominance Hierarchy: T > t
The T-Locus is responsible for patterns of pigmented flecks which may appear on areas that are 'white spotted', which are as you now know controlled by the S-Locus. The most common manifestation of these flecks in Border Collies is called 'ticking' where the pigment shows clearly as small spots, however 'roaning' which is seen in other breeds such as Australian Cattle Dogs and Cocker Spaniels is also a result of the alleles at the T-Locus, where the pattern of pigment is heavily mottled and often only a small amount of scattered white is visible. Regular ticking looks like coloured spots on white, whereas roaning looks like little spots of white on colour. Ticking is generally seen more frequently and at a higher density in working lines, however is still quite prominent in some show lines and is not a fault.
Dalmatian spots are also a form of ticking, with another genetic modifier acting upon them so the spots are bigger than regular ticking, sparser, and more evenly distributed. Dogs are not born with visible ticking; it develops over time which is most noticeable in breeds such as Dalmatians and Australian Cattle Dogs who are born white (ACDs have head markings and potentially some body patches) with their ticking (spots)/roaning (mottled colour) developing later. In Border Collies ticking works in the same way with the spots not usually visible at birth and developing later on white areas, however Border Collie pups themselves are not born white (unless there are other genetic variants such as extreme white or double merle at play) as their coat colours are more dependent on the other genetics covered previously rather than ticking.
Ticking flecks will be the colour that the coat in that area would otherwise have been, had it not been white. This means for a tricolour dog that ticking on the body would be the respective base colour however ticking on white areas of the muzzle, chest or legs where tan points would usually be, would instead be tan. Generally, ticking is heaviest on the muzzle and legs and this is where it will most likely show up in a dog with light ticking. Ticking on a toe can also have an effect on the toenail, so some dogs may have one or more black nails while the rest are white - it is all dependent on the location of the ticking. If a dog has the ticking allele but no white for it to show up on, there will be no visible effect. It can also be tricky to distinguish on a merle or piebald dog, where the pigment is already quite mottled, however as already stated merle acts on the base coat at the B-Locus or D-Locus, while ticking will only show up on white areas caused by the S-Locus. The giveaway is usually that a merle dog will probably still have white in all the usual places. The ticking gene (T) is thought to be dominant however the amount and density of ticking can vary greatly and likely be explained by incomplete dominance (explained further below). Roaning has previously been thought to be very heavy ticking, however research appears to be showing that it could be attributed to a separate allele (Tr).
Known Alleles:
T - Ticking - the dog will have ticking (small flecks) to some degree on white areas, most likely the muzzle and legs.
t - No Ticking - pigment spots will not be produced.
Theoretical Allele:
Tr - Roaning - responsible for large clusters of spots which almost look like the white is peeking through the colour.
As with the A-Locus where the sable and tanpointed genes are controlled, the T-Locus is also subject to incomplete dominance where homozygous (same) pairs will exhibit the phenotype as expected however a heterozygous (different) pair will mostly exhibit the dominant characteristics however also show some effect of the recessive allele as well.
Therefore, the possible basic genotypes and phenotypes that can be produced are as follows:
TT - Homozygous Dominant Ticking - the dog is likely heavily ticked on white areas
Tt - Heterozygous Ticking - the dominant ticking is expressed, but perhaps to a lesser degree
tt - Homozygous Recessive No Ticking - white areas stay white
Dalmatian spots are also a form of ticking, with another genetic modifier acting upon them so the spots are bigger than regular ticking, sparser, and more evenly distributed. Dogs are not born with visible ticking; it develops over time which is most noticeable in breeds such as Dalmatians and Australian Cattle Dogs who are born white (ACDs have head markings and potentially some body patches) with their ticking (spots)/roaning (mottled colour) developing later. In Border Collies ticking works in the same way with the spots not usually visible at birth and developing later on white areas, however Border Collie pups themselves are not born white (unless there are other genetic variants such as extreme white or double merle at play) as their coat colours are more dependent on the other genetics covered previously rather than ticking.
Ticking flecks will be the colour that the coat in that area would otherwise have been, had it not been white. This means for a tricolour dog that ticking on the body would be the respective base colour however ticking on white areas of the muzzle, chest or legs where tan points would usually be, would instead be tan. Generally, ticking is heaviest on the muzzle and legs and this is where it will most likely show up in a dog with light ticking. Ticking on a toe can also have an effect on the toenail, so some dogs may have one or more black nails while the rest are white - it is all dependent on the location of the ticking. If a dog has the ticking allele but no white for it to show up on, there will be no visible effect. It can also be tricky to distinguish on a merle or piebald dog, where the pigment is already quite mottled, however as already stated merle acts on the base coat at the B-Locus or D-Locus, while ticking will only show up on white areas caused by the S-Locus. The giveaway is usually that a merle dog will probably still have white in all the usual places. The ticking gene (T) is thought to be dominant however the amount and density of ticking can vary greatly and likely be explained by incomplete dominance (explained further below). Roaning has previously been thought to be very heavy ticking, however research appears to be showing that it could be attributed to a separate allele (Tr).
Known Alleles:
T - Ticking - the dog will have ticking (small flecks) to some degree on white areas, most likely the muzzle and legs.
t - No Ticking - pigment spots will not be produced.
Theoretical Allele:
Tr - Roaning - responsible for large clusters of spots which almost look like the white is peeking through the colour.
As with the A-Locus where the sable and tanpointed genes are controlled, the T-Locus is also subject to incomplete dominance where homozygous (same) pairs will exhibit the phenotype as expected however a heterozygous (different) pair will mostly exhibit the dominant characteristics however also show some effect of the recessive allele as well.
Therefore, the possible basic genotypes and phenotypes that can be produced are as follows:
TT - Homozygous Dominant Ticking - the dog is likely heavily ticked on white areas
Tt - Heterozygous Ticking - the dominant ticking is expressed, but perhaps to a lesser degree
tt - Homozygous Recessive No Ticking - white areas stay white
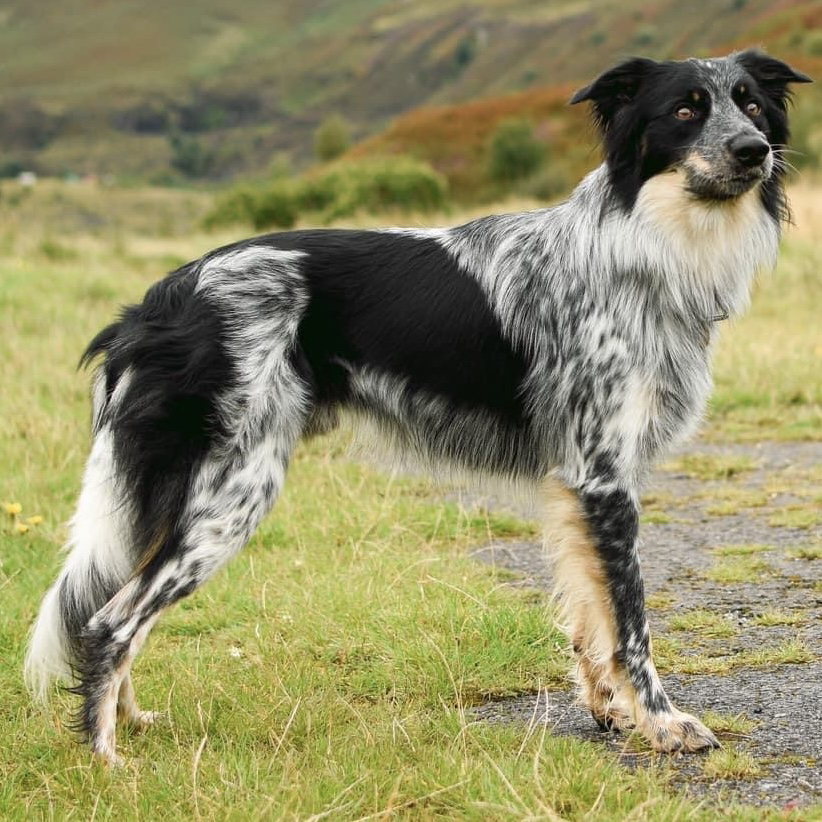
A piebald black tricolour dog with such heavy ticking/roaning some would mistake him for merle - it is the ticking on what would otherwise be white areas that most easily clarifies otherwise, however DNA testing is the only way to truly confirm all coat colour genotypes. Note how the colour of the ticking varies based on his tan points, and is so heavily concentrated on the muzzle that it almost completely covers the white blaze.
Photo of ‘Boomerang’ courtesy of Jessica Leigh, Cardiff (Wales).
© Border Collie Club of South Australia Inc. 2025